Best CNC Methods & Tools (Drill Bits) for Stainless Steel Machining
Machining stainless steel presents unique challenges due to its material properties, which can lead to common issues such as tool sticking, burning, or breakage. These problems often occur because of inadequate tool selection, improper machining methods, or a lack of understanding of the material’s characteristics. This article will provide a detailed, professional overview of the best practices for machining stainless steel, focusing on optimizing machining methods and selecting the most appropriate tools to achieve higher precision, longer tool life, and improved productivity.
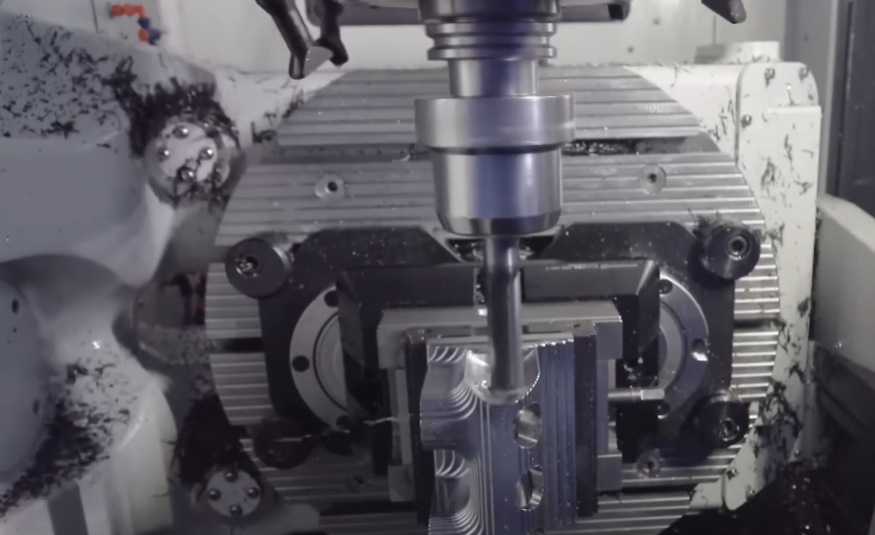
1. Classification and Characteristics of Stainless Steel
Stainless steel is an alloy primarily composed of iron, with added elements such as chromium (Cr), nickel (Ni), manganese (Mn), titanium (Ti), and molybdenum (Mo) to enhance its properties. The alloying elements give stainless steel its well-known corrosion resistance, oxidation resistance, and high-temperature strength, making it ideal for various demanding applications.
However, these same properties also introduce challenges during machining. Due to its high strength, stainless steel generates significant cutting forces, resulting in rapid tool wear. Additionally, its low thermal conductivity causes heat to accumulate in the cutting zone, especially around the cutting edges, which accelerates wear and can lead to tool failure. Moreover, stainless steel is prone to work hardening, meaning that the surface hardens during machining, making subsequent cutting passes more difficult.
Stainless steel is generally divided into four main types based on its microstructure:
- Austenitic Stainless Steel: The most commonly used type, known for its excellent corrosion resistance and ductility. Examples include grades 304 and 316. It is non-magnetic and has high toughness but is also prone to work hardening.
- Martensitic Stainless Steel: This type offers higher strength and hardness due to its martensitic structure but has lower corrosion resistance compared to austenitic grades. It is often used in applications requiring high wear resistance, such as cutlery and surgical instruments.
- Precipitation-Hardening Stainless Steel: This type combines high strength and moderate corrosion resistance, achieved through heat treatment. It is used in aerospace and other high-stress applications.
- Duplex Stainless Steel: A combination of austenitic and ferritic structures, duplex stainless steel offers higher strength and better stress-corrosion cracking resistance than austenitic grades, making it suitable for chemical processing and offshore applications.
Each type of stainless steel has unique machining characteristics, which require careful consideration of cutting parameters, tool selection, and machining strategies.
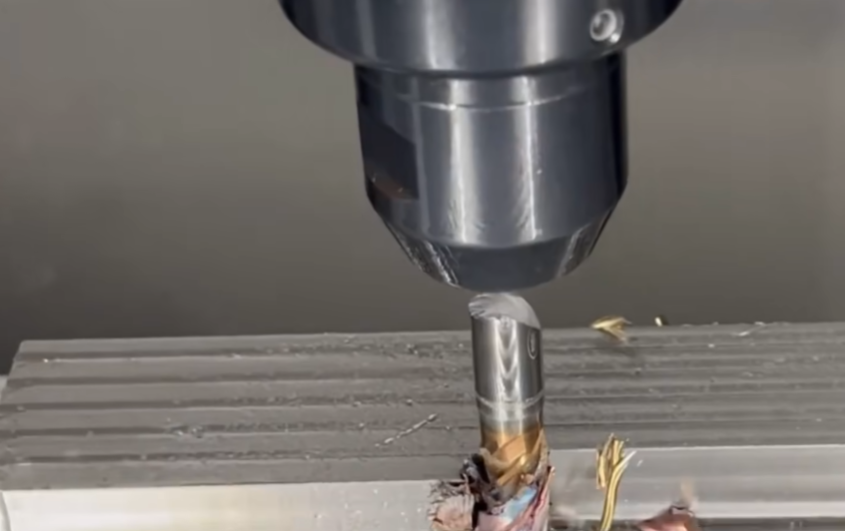
2. Optimizing Machining Methods for Stainless Steel
To successfully machine stainless steel, it is essential to address its specific thermal and mechanical properties. The following machining strategies can help mitigate the challenges posed by this material:
2.1. Heat Management and Cooling
Effective heat management is critical when machining stainless steel due to its poor thermal conductivity. Excessive heat in the cutting zone can cause tool wear, dimensional inaccuracies, and thermal expansion of the workpiece. Therefore, implementing efficient cooling strategies is paramount:
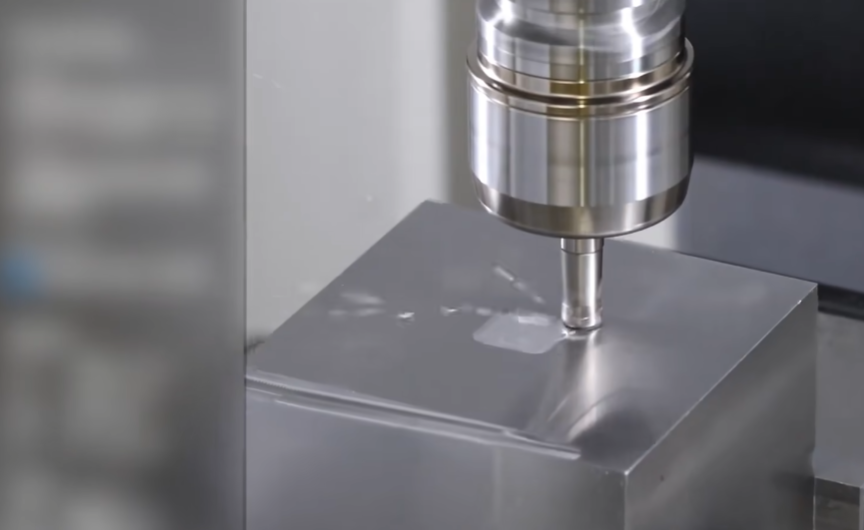
- Flood Cooling: Utilizing a high-volume flow of coolant directly onto the cutting zone can help dissipate heat quickly. This is especially important in high-speed cutting operations. The coolant should be applied to both the cutting edge and the workpiece to prevent localized heating.
- High-Pressure Internal Cooling: For deep hole drilling or high-feed milling, it is recommended to use high-pressure coolant systems that deliver coolant through the spindle and directly to the cutting edge. This ensures that the cutting zone remains cool and chips are flushed away effectively.
- Mist or MQL (Minimum Quantity Lubrication) Cooling: In some cases, mist cooling or MQL can be used to reduce the amount of coolant while still providing effective lubrication and cooling. This method can be particularly useful in high-speed, dry machining applications where traditional flood cooling might not be feasible.
2.2. Chip Control and Evacuation
Efficient chip control is another critical aspect of stainless steel machining. Due to the material’s toughness and ductility, chips tend to form long, continuous ribbons, which can interfere with the cutting process if not properly managed:
- Chip Breakers: Ensure the tool has an optimized chip breaker design to control chip formation and break up chips into smaller, more manageable segments. This will help prevent chip entanglement and reduce the likelihood of damaging the workpiece or tool.
- Chip Evacuation: In operations like turning, boring, or drilling, rapid chip evacuation is essential to avoid chip re-cutting, which can lead to poor surface finish and accelerated tool wear. Tools with internal coolant channels can help flush chips away from the cutting zone, improving both tool life and machining efficiency.
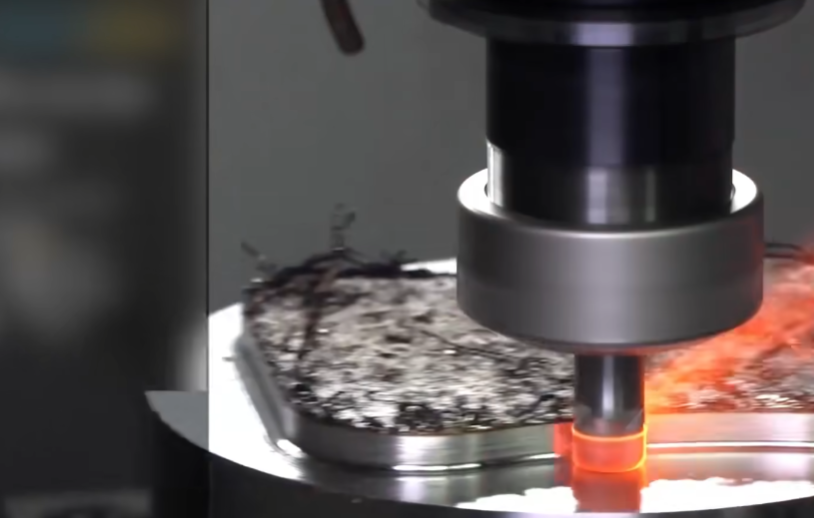
3. Tool Selection for Stainless Steel Machining
The choice of cutting tools plays a critical role in determining the success of stainless steel machining operations. Since stainless steel is more abrasive and work-hardening than many other metals, tool material, geometry, and coatings must be carefully selected.
3.1. Tool Materials
- Carbide Tools: Cemented carbide tools are the preferred choice for stainless steel machining due to their high hardness and wear resistance at elevated temperatures. Carbide tools can withstand the higher cutting forces and heat generated during stainless steel machining, making them ideal for both roughing and finishing operations.
- Cermet and Ceramic Tools: In high-speed machining of martensitic or precipitation-hardening stainless steels, cermet and ceramic tools can sometimes be used effectively. However, these tools are more brittle than carbide and may require more precise control of cutting parameters.
- High-Speed Steel (HSS) Tools: HSS tools are less commonly used for stainless steel due to their lower wear resistance compared to carbide. However, they may still be suitable for less demanding operations, such as drilling or tapping, where carbide is too brittle.
3.2. Tool Coatings
Tool coatings are essential for improving tool life and performance in stainless steel machining. Common coatings include:
- PVD (Physical Vapor Deposition) Coatings: PVD coatings, such as titanium aluminum nitride (TiAlN) or chromium nitride (CrN), are highly recommended for stainless steel machining. These coatings provide excellent heat resistance and wear protection, reducing friction and preventing built-up edge formation.
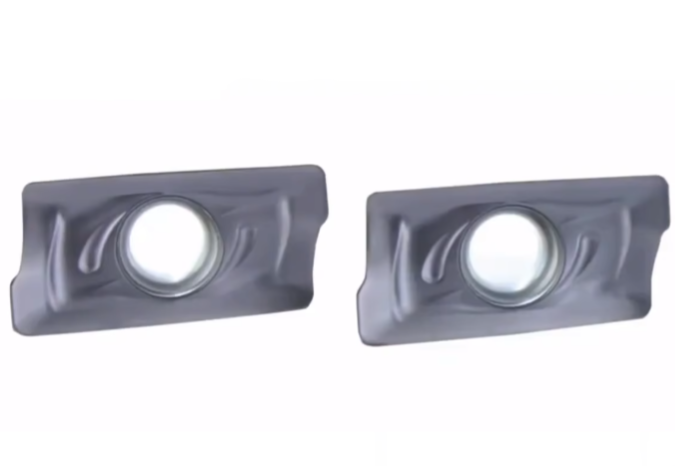
- CVD (Chemical Vapor Deposition) Coatings: CVD coatings, such as titanium carbide (TiC), are more wear-resistant but can be thicker and less suitable for applications where sharp cutting edges are required.
- Diamond-Like Carbon (DLC) Coatings: These coatings are highly effective in reducing friction and preventing adhesion between the tool and the workpiece, making them useful for high-feed applications.
3.3. Tool Geometry
Tool geometry is a crucial factor in optimizing stainless steel machining. The following features should be carefully considered:
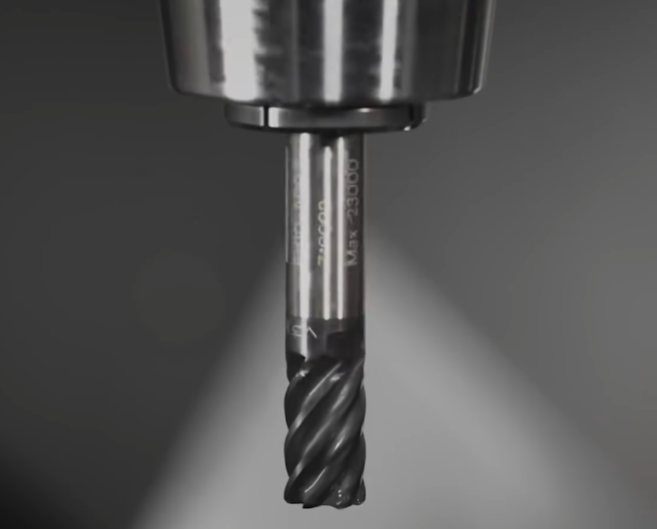
- Rake Angle: A positive rake angle helps reduce cutting forces, making it easier to cut through tough stainless steel alloys. A larger rake angle also helps reduce tool wear and improves surface finish by minimizing the build-up of material on the cutting edge.
- Relief Angle: The relief angle should be optimized to ensure adequate clearance between the tool and the workpiece. An insufficient relief angle can lead to rubbing and increased heat generation, while an overly large relief angle can weaken the cutting edge.
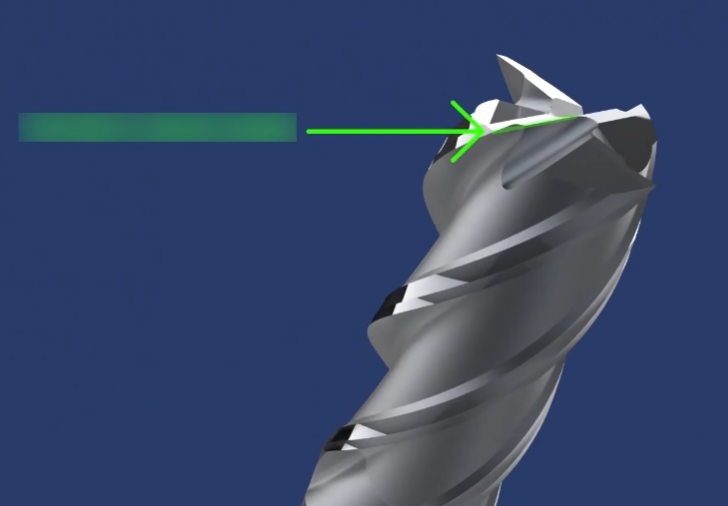
- Cutting Edge Inclination: A positive cutting edge inclination promotes smoother chip flow and reduces the likelihood of chip adhesion to the tool. In finishing operations, a positive cutting edge inclination can help reduce surface damage caused by chip re-cutting.
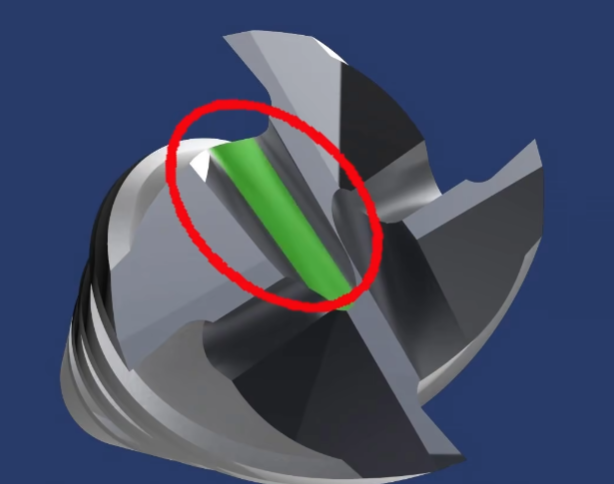
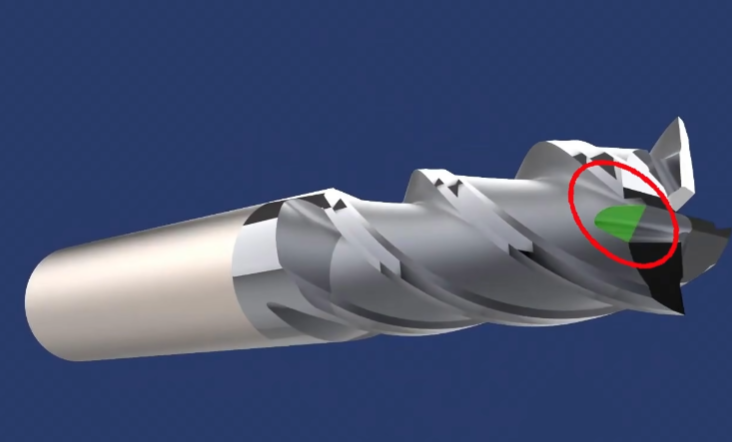
- Lead Angle: A larger lead angle can distribute cutting forces more evenly across the cutting edge, improving tool life and surface finish. This is particularly useful in operations like turning and milling.
4. Optimizing Cutting Parameters for Stainless Steel
Selecting the right cutting parameters is essential for maximizing productivity and tool life in stainless steel machining. The following guidelines can help optimize cutting conditions:
4.1. Cutting Speed
Stainless steel has a lower thermal conductivity than many other metals, meaning that heat generated during machining tends to concentrate in the cutting zone. This requires the use of lower cutting speeds to maintain tool life and prevent overheating. For austenitic stainless steel, typical cutting speeds range from 60 to 150 meters per minute (m/min) for carbide tools, depending on the specific alloy and operation.
4.2. Feed Rate
The feed rate should be moderate to high to prevent work hardening, which can occur when the tool lingers in the same area for too long. A higher feed rate also helps prevent the formation of built-up edge (BUE), which can damage the tool and degrade the surface finish.
4.3. Depth of Cut
The depth of cut should be sufficient to penetrate any surface-hardened layer, especially in materials like austenitic stainless steel that are prone to work hardening. In general, a depth of cut of 0.1 to 0.3 mm for finishing and 0.5 to 2 mm for roughing is recommended.
With these approaches, you can achieve consistent, high-quality results in stainless steel machining, even in the most demanding applications.